Educational aims and objectives
This self-instructional course for dentists aims to discuss how the soft tissue laser can be applied in dental surgery and implant procedures.
Expected outcomes
Implant Practice US subscribers can answer the CE questions by taking the quiz to earn 2 hours of CE from reading this article. Correctly answering the questions will demonstrate the reader can:
- Realize how different types of lasers are absorbed by biological tissues.
- Recognize that efficient decontamination of the implant surfaces can be achieved without heating or damaging the implant.
- Realize how the depth of the incision is controlled with the laser, so the clinician can adjust technique for a very wide range of applications.
- Identify how laser light can affect safe decontamination of the implant surfaces.
- Realize how the laser affects the depth of coagulation/hemostasis.
- Observe how CO2 laser surgery can result in reduced scarring and reduced postoperative pain.
Peter Vitruk discusses how a SuperPulse laser can be integral to the success of soft tissue surgery.
Peter Vitruk, PhD, MInstP, CPhys, sheds some light on the many applications of lasers
Laser light absorption by the biological tissues — ablative and nonablative lasers
The key to the success of lasers in soft tissue surgery is their ability to (a) cut and (b) coagulate the soft tissue at the same time. Not all laser wavelengths are suitable to comply with both requirements.
To understand how laser light cuts and coagulates the soft tissue, one must consider how the laser light is absorbed and scattered by the soft tissue.1-3 Most commercially available dental lasers fit into three wavelength categories:
- Near-infrared circa 1,000 nm (810-1,064 nm diodes and 1,064 nm Nd-laser)
- Mid-infrared circa 3,000 nm (2,790 and 2,940 nm Er-lasers)
- Infrared circa 10,000 nm (9,300 and 10,600 nm CO2 lasers)
The absorption spectra of oral soft tissue are summarized in Figure 1 along with Ablation Thresholds and Coagulation Depths for popular soft tissue dental lasers.
Because near-infrared (Near-IR) photons are weakly absorbed (1,000-10,000 times weaker than CO2 and Erbium), dental diodes are not used to cut soft tissue with photons. Instead, dental diodes cut soft tissue thermomechanically with hot charred glass tips, whereas the tip-tissue contact time defines the depths of its thermal impact. These diode laser wavelengths, however, are scattered much stronger than they are absorbed, resulting in a strong and widespread Near-IR halo of light underneath the irradiated surface of the soft tissue.3 These Near-IR diode laser wavelengths are referred to as nonablative, as opposed to ablative Erbium and CO2 laser wavelengths, which are weakly scattered but strongly absorbed by the soft tissue.
Mid-IR Erbium laser wavelengths are highly energy-efficient and spatially accurate photothermal ablation tools, but with an insufficient depth of coagulation (significantly less than blood capillary diameters).
IR CO2 laser wavelengths are highly efficient and spatially accurate photothermal ablation tools with good coagulation efficiency due to a close match between coagulation depth and oral soft tissue blood capillary diameters.
SuperPulse and Thermal Relaxation Time
Thermal Relaxation Time (TRT) is a measure of how fast the irradiated tissue diffuses the heat into the adjacent tissues.3,4 The most efficient heating of the irradiated tissue takes place when the laser pulse duration is shorter than the TRT. The most efficient cooling of the tissue adjacent to the ablated zone takes place if the time duration between laser pulses is greater than the TRT. Such laser pulse design in CO2 lasers is referred to as SuperPulse,3 depicted in Figure 2. SuperPulse minimizes the amount of heat that is diffusing away from the cutting/ablation zone to the adjacent healthy tissue.
SuperPulse CO2 laser removal of biofilms from dental implant surfaces
Efficient decontamination of the implant surfaces can be achieved by the SuperPulse CO2 laser beam5,6 without heating or damaging the implant7,8 for the following reasons:
- This 10,600 nm wavelength is highly efficiently absorbed by water-rich bacterial biofilms on the implant surface (Figure 1).
- The short pulse duration of the SuperPulse allows for highly efficient confinement of the laser-
generated heat inside the biofilm without thermal conduction of heat away into the implant (Figure 2). - SuperPulse’s high peak power allows for fast vaporization (ablation) of biofilms since the laser-generated heat inside the biofilm is sufficient for vaporizing it.
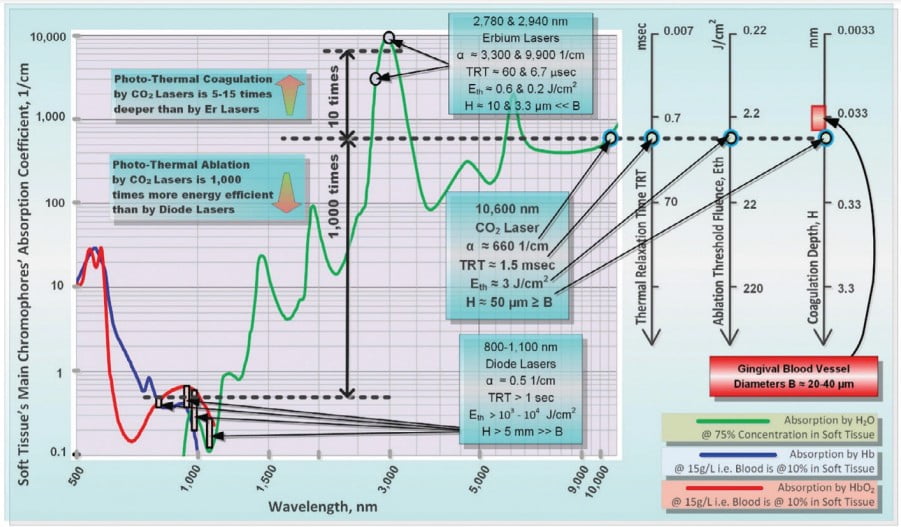
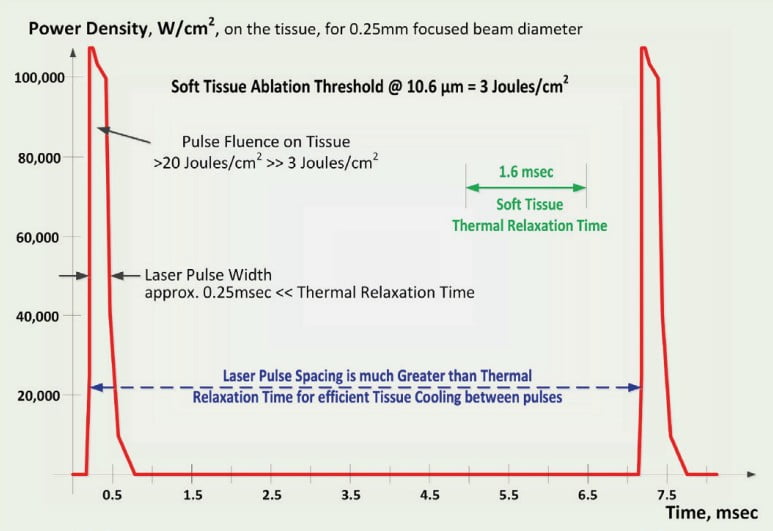
The SuperPulse 10.600 nm CO2 laser treatment with an average fluence in excess of 40 J/cm2 provides a 100% removal of an in vitro biofilms of approximately 10 µm thickness grown on titanium and titanium-oxide implant specimens with a moderately rough surface topography.6
Such CO2 laser irradiation of the titanium implant surface during open flap procedures allows for high success outcomes9 when treating peri-implantitis as illustrated in Figure 3.
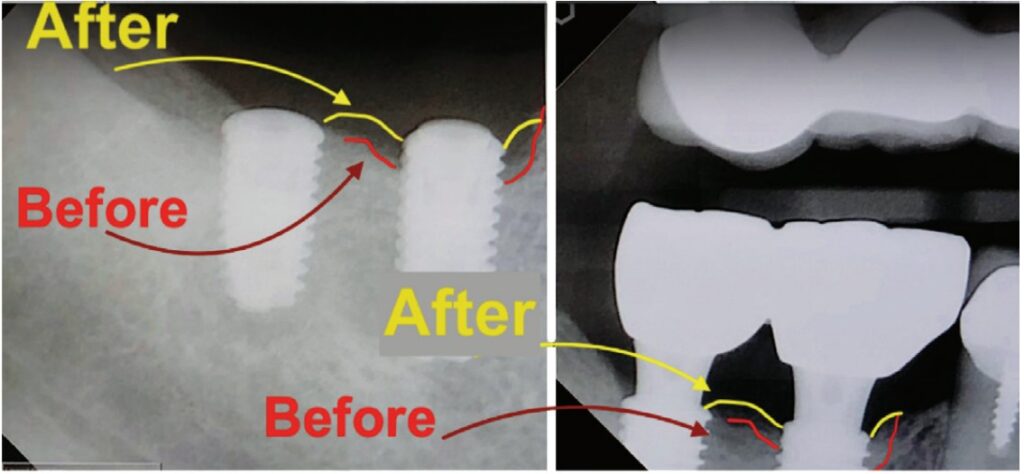
Implant-Safe Lasers — Implant-Safe SuperPulse CO2 Laser settings
Safe decontamination of implant surfaces with laser light can be achieved if the laser energy is efficiently reflected off the implant surface after the diseased tissue and biofilms are vaporized (ablated) from the implant surface.6-8
This condition is easily met for titanium implants.6-8 As illustrated in Figure 4, the 10.6 micrometer CO2 laser wavelength is highly reflected (>90%) from titanium.10 Diode, Nd-laser, and Er-laser wavelengths are not as efficiently reflected and produce 3 to 4 times greater rate of the implant heating than the CO2 laser for a comparable dose of laser energy. This property makes the CO2 laser wavelength the safest available wavelength for peri-implantitis treatment.
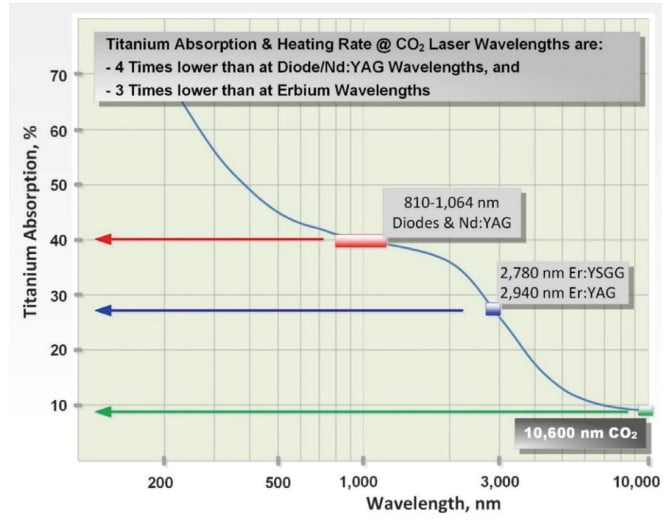
Titanium implants (Biomet 3i NanoTite™ NIITP4310 and Biomet 3i Osseotite™ IFNT510 — all are trade names and trademarks of Biomet) have been demonstrated8 to safely handle a SuperPulse CO2 laser’s average fluence in 320 J/cm2 –360 J/cm2 range, which are much greater fluence values than those proven efficient for biofilm ablation (> 40 J/cm2).6 It has been also demonstrated in the same study8 that SuperPulse CO2 laser irradiation of these implants produces significantly less heat when compared to the diode laser wavelengths.
Soft tissue surgery and incision/ablation depth with laser beams
For the ablative CO2 and Erbium lasers, the power density of the focused laser beam is equivalent to the mechanical pressure that is applied to a cold steel blade.11 Greater laser fluence (i.e., greater power density and slower hand speed) results in a greater depth and rate of soft tissue vaporization. More specifically, as illustrated in Figure 5, the depth of incision is proportional to laser beam average power and inversely proportional to laser beam spot size and the surgeon’s hand speed.
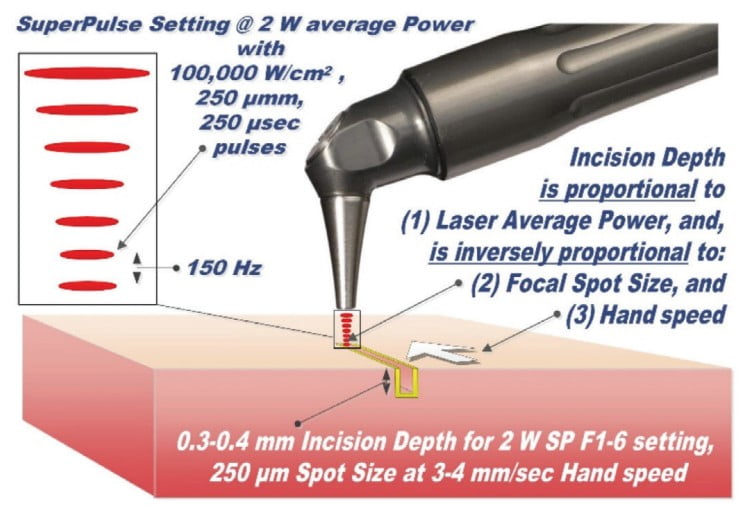
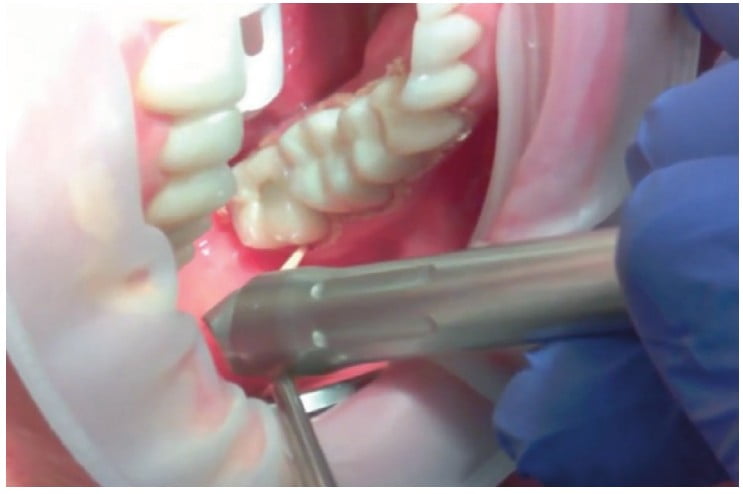
Understanding how the depth of the incision is controlled through power, spot size, and hand speed, a skilled dental surgeon can easily adjust the technique for a very wide range of applications. When decontaminating the dental implant surfaces described earlier, the depth of the biofilms removed is under 20 micrometers. During the closed-flap sulcular debridement with the CO2 laser, the diseased epithelium can be removed12 with similar micrometer-range precision and accuracy as illustrated in Figure 6. In certain popular soft tissue applications — e.g., lingual frenectomy13 — the skilled laser user can carefully debride the fibrous tissue even from around blood vessels if need be to fulfill the complete release of restrictions without puncturing the exposed blood vessels as illustrated in Figures 7 and 8.
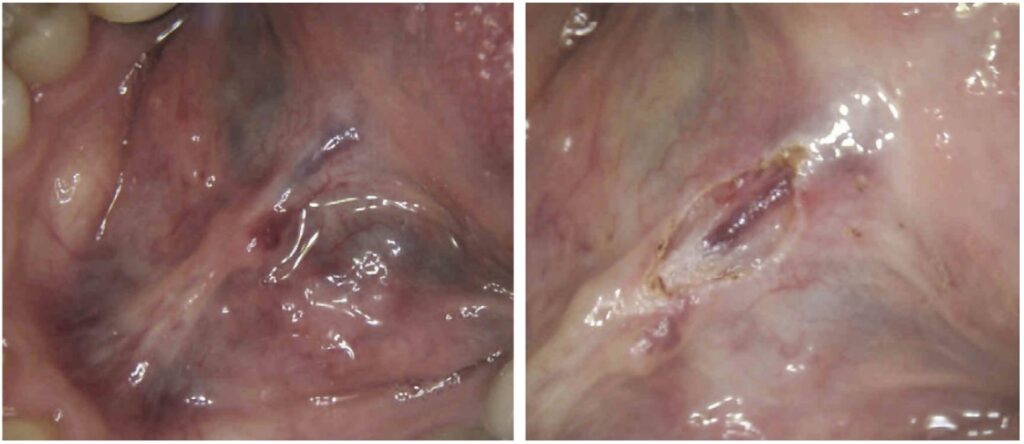
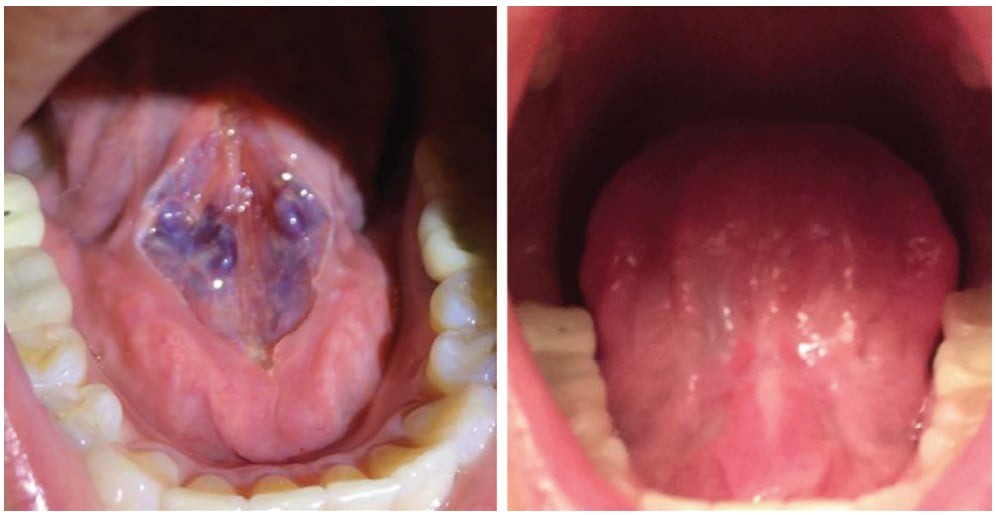
Depth of coagulation/hemostasis with laser beams
Coagulation occurs as a denaturation of soft tissue proteins that takes place in the 60°C to 100°C temperature range3 leading to a significant reduction in bleeding (and oozing of lymphatic liquids) on the margins of ablated tissue. Photothermal coagulation is also accompanied by hemostasis due to shrinkage of the walls of blood and lymphatic vessels due to collagen shrinkage at increased temperatures. Since blood is contained within and transported through the blood vessels, the diameter of blood vessels B (20-40 µm from measurements in human cadaver gingival tissue16) is a highly important spatial parameter in considering the efficiency of photothermal coagulation.
For diode laser wavelengths, optical absorption (Near-IR attenuation) and coagulation depths are significantly greater than blood vessel diameters; coagulation takes place over extended volumes, extending far beyond ablation margins where no coagulation is required.3,11,17
For pulsed lasers (e.g., SuperPulse CO2 and free-running pulse Erbium lasers) the coagulation depth is proportional to the absorption depth of light in the soft tissue. The depth of coagulation/hemostasis can be extended by longer and lower power laser pulses, which allow the heat to diffuse away from the irradiated superficial depths into the deeper regions of the target tissue. 3,11
For Erbium laser wavelengths, the optical absorption depth and the coagulation depth are significantly smaller than blood vessel diameters (Figure 1); coagulation takes place on a relatively small spatial scale and cannot prevent bleeding from the blood vessels severed during efficient tissue ablation.3,11
For CO2 laser wavelengths (Figure 1), sub-100 µm5,7 coagulation extends just deep enough into a severed blood vessel to stop the bleeding; the coagulation and hemostasis are more efficient than for Erbium laser wavelengths.3,11
Figures 6-10 illustrate great hemostasis and coagulation on surgical margins during and immediately after the CO2 laser surgery.
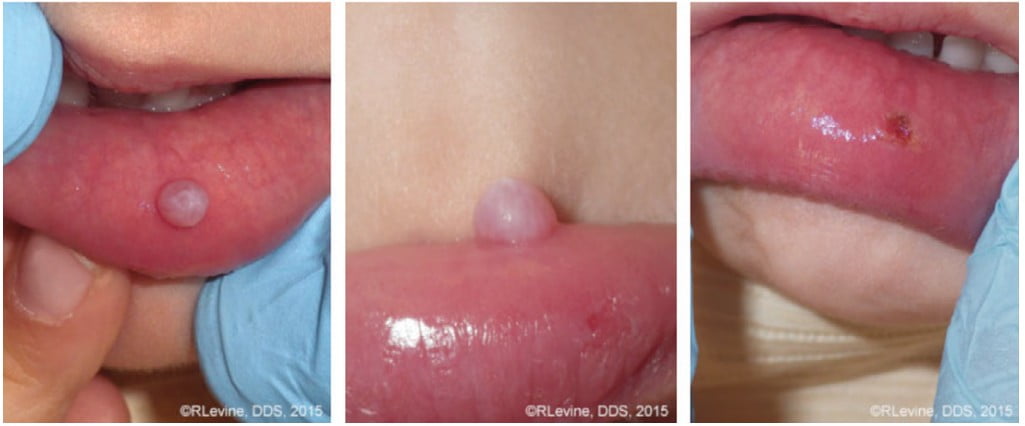
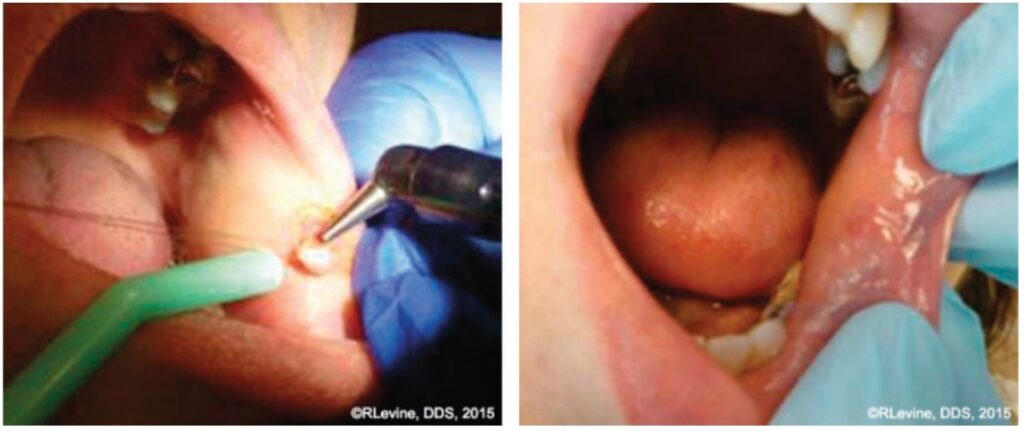
Reduced scarring and reduced postoperative pain following CO2 laser surgery18
Both the reduced scarring and postoperative pain can be partially explained by the optimal coagulation/hemostasis depth on CO2 laser surgical margins. Several studies have found the reduced presence of contractile myofibroblasts — cells accountable for postoperative scarring — in CO2 laser surgical wounds when compared to scalpel surgery, which explains the lesser postoperative scarring following CO2 laser surgery. Also, decreased extravasation of blood and lymphatic fluids into the CO2 laser wound space impedes the release of inflammatory mediators. This results in less edema around the wound then following conventional surgery and delayed minimal inflammatory response. It may also account for the reduced immediate postoperative pain after CO2 laser surgery. Despite the abundant published case studies and research as well as anecdotal reports regarding reduced pain following CO2 laser surgery, the exact mechanism behind it remains to be explained.
Understanding how the depth of the incision is controlled through power, spot size, and hand speed, a skilled dental surgeon can easily adjust the technique for a very wide range of applications.
Nonablative lasers and near-infrared diode hot glass tip
As previously explained and further illustrated in Figure 11, the near-IR laser wavelengths of diode lasers are weakly absorbed by the soft tissue and cannot radiantly ablate/vaporize the soft tissue3,11,17-21 (except for high melanin content epithelium).
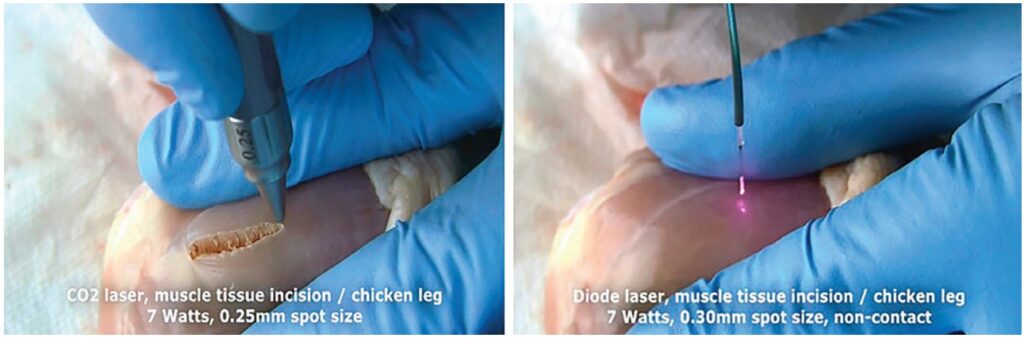
Since diode Near-IR laser wavelengths are not suitable for the oral soft-tissue cutting, there is a different technique of non-optical (non-radiant) tissue cutting with the so-called “hot tip,”19-21 as illustrated in Figure 12.
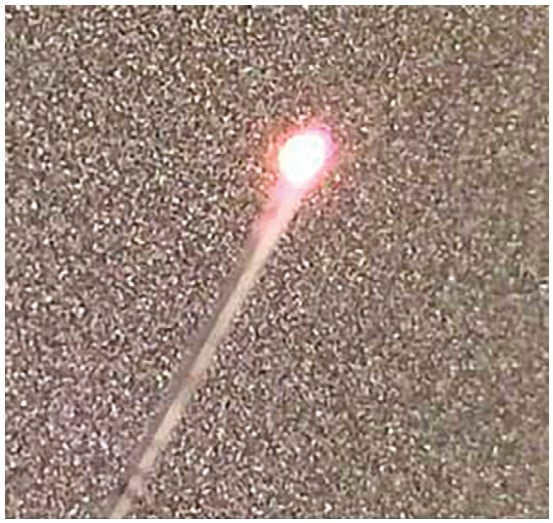
A critical component of such hot tip-tissue interaction is the creation of the optically dark char deposit on the very end of the glass tip. The diode laser optical energy is then used to heat the charred glass fiber’s tip up to 1,000ºC to 1,500ºC.19-21 The hot glass tip heats soft tissue through heat conduction (i.e., heat diffusion) from (and through) hot glass tip to (and through) the soft tissue. Charred glass tip diode lasers perform as non-laser wavelength-independent ablation thermal devices with coagulation depths in the range 250-1,000 µm22 (similarly to electrocautery where soft tissue is cut by the hot metal tip). The cutting speed of the charred hot glass tip is limited by its disintegration at elevated temperatures (up to 1,500ºC), thus raising concerns about biocompatibility19 of the burnt tip’s cladding chemicals and thermally fractured glass.
Degradation of the char on glass tip surface creates the “optically leaky” tip with a reduced tip temperature, and increased risk of Near-IR induced sub-surface thermal necrosis20 as well as mechanical tearing of the tissue by the cold glass’ sharp edges, which may result in both deep thermal trauma and excessive superficial bleeding as illustrated in Figure 13.
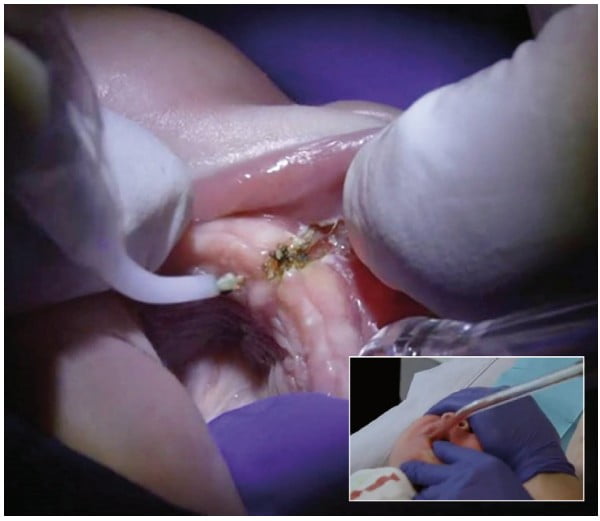
ANSI Z136.3 Standard for Safe Use of Lasers in Health Care
Laser Institute of America (LIA) has originally developed and now regularly updates the Laser Safety regulations (ANSI Z136.3 Standard for Safe Use of Lasers in Health Care – 2018 Edition23), which are mandatory for implementation by all laser users in mitigating laser beam and non-beam hazards.
The most important top-level Laser Hazard mitigation measures are typically well understood and properly implemented by the majority of the surgical laser users — i.e., Laser Safety Officer (LSO) appointment, Laser In Use Sign, and Laser Safety Goggles Administration. Additionally, the ANSI Z136.3 Standard defines laser plume as one of the non-beam laser hazards since it contains viral, bacterial, and other cellular and aerosolized particulates, gaseous toxic compounds, etc.
ANSI Z136.3 Standard specifies safety measures to mitigate the laser plume hazards, i.e., the mandatory use of Local Exhaust Ventilation (LEV) device — for example, wall suction devices in dental offices (aka high-volume evacuation devices and mobile/portable smoke evacuators in physicians’ and veterinarians’ offices) — equipped with a proper filter (with ANSI Z136.3 Standard’s defined filtration at 0.12 µm at 99.999% efficiency) and with wide aperture suction nozzle held as close as possible to the surgical site (no further than 2 inches per the CDC24 — Figure 14) to safely remove laser plume.
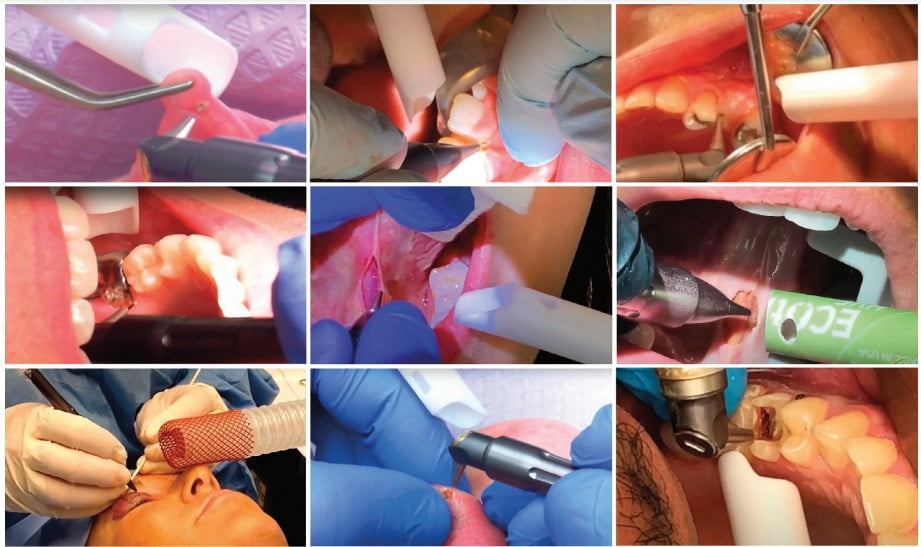
According to ANSI Z136.3(2018), the use of N95 is preferred over surgical masks, but only in addition to laser plume evacuation.
It is important to emphasize that in surgical laser uses that do not utilize water sprays, laser plume can be safely removed according to and in compliance with ANSI Z136.3 Standard. However, users of dental lasers that utilize water sprays should be aware that ANSI Z136.3 Standard does not address the safe removal of the laser plume dispersed by the water spray.25
References
- Jacques SL. Origins of tissue optical properties in the UVA, visible, and NIR regions. In: Alfano RR, Fujimoto JG (ed.) OSA TOPS on Advances in Optical Imaging Photon Migration. Optical Society of America. 1996.
- Jacques SL. Optical properties of biological tissues: a review. Phys Med Biol. 2013; 58(11):R37-61.
- Vitruk P. Oral soft tissue laser ablative and coagulative efficiencies spectra. Implant Practice US. 2014;7(6):19-27.
- Vogel A, Venugopalan V. Mechanisms of pulsed laser ablation of biological tissues. Chem Rev. 2003;103(2):577-644.
- Romanos GE, Ko HH, Froum S, Tarnow D. The use of CO2 laser in the treatment of peri-implantitis. Photomed Laser Surg.2009;27(3):381-386.
- Cobb CM, Vitruk P. Effectiveness of a super-pulsed CO2 laser for removal of biofilm from three different types of implant surfaces: an in vitro study. Implant Practice US.2015;8(3):20-28.
- Linden E, Vitruk P. SuperPulse 10.6 µm CO2 laser-assisted, closed flap treatment of peri-implantitis.Implant Practice US. 2015;8(4):34-38.
- Linden E, Vitruk P. Implant-safe settings for SuperPulse 10.6 micrometers CO2 laser-assisted, closed flap peri-implantitis treatment. Implant Practice US. 2015;8(6):26-28.
- Shulman M. CO2 Laser Peri-Implantitis Treatment Results. https://www.youtube.com/watch?v=bV8dfT6I17g&list=PL4ROT89t1sVtyFCoK6Gls4E0xqKlSOvbm&index=70
- Wolfe WL, Zissis GJ. The Infrared Handbook. Office of Naval Research. 1985:7-81.
- Riek C, Vitruk P. Incision and Coagulation/Hemostasis Depth Control During a CO2 Laser Lingual Frenectomy. Dental Sleep Practice. spring2018;32-38.
- Sutter BA. Identification and Laser Eradication of Oral Cavity Spirochetes. Case Study. 2018;1(1):4-13. https://www.youtube.com/watch?v=GboQ3Y7h0eQ&list=PL4 ROT89t1sVtyFCoK6Gls4E0xqKlSOvbm&index=40. Accessed January 21, 2022.
- Kaplan M, Vitruk P. Soft tissue 10.6 micrometers CO2 laser orthodontic procedures. Orthodontic Practice US.2015;6(6):53-57.
- Levine R, Vitruk P. Laser Mucocele Removal in Pediatric Patients. 2016;17(9):10-111.
- Levine R, Vitruk P. Enhanced hemostasis and improved healing in CO2 laser-assisted soft tissue oral surgeries. Implant Practice US.2015;8(3):34-37.
- Yoshida S, Noguchi K, Imura K, Miwa Y, Sunohara M, Sato I. A morphological study of the blood vessels associated with periodontal probing depth in human gingival tissue. Okajimas Folia Anat Jpn. 2011;88(3):103-109.
- Willems PWA, Vandertop WP, Verdaxsasdonk RM, van Swol CFP, Jansen GH. Contact laser-assisted neuroendoscopy can be performed safely by using pretreated ‘black’ fibre tips: Experimental data. Lasers Surg Med. 2001;28(4):324-329.
- Glazkova A, Vitruk P. CO2 Laser Surgery Post-Operative Pain and Healing: A Partial Literature Review. Dental Sleep Practice. Winter 2019:28-34.
- Vitruk P. Laser Education, Science and Safety – A review of dental laser education standards. 2017;17(6):62-67.
- Vitruk P. Hot Glass Tip Diode Frenectomies ARE NOT Laser Frenectomies. Dental Sleep Practice US. Summer 2019;48-50.
- Romanos GE. Diode Laser Soft-Tissue Surgery. Compend Contin Educ Dent. 2013;34(10):752-757
- Vitruk P, Levine R. Hemostasis and Coagulation with Ablative Soft-Tissue Dental Lasers and Hot-Tip Devices. Inside Dentistry. 2016;12(8):37-42.
- The Laser Institute of America. American National Standards Institute – ANSI Z136.3. Safe Use of Lasers in Health Care. https://www.lia.org/resources/laser-safety-information/laser-safety-standards/ansi-z136-standards/z136-3. Accessed January 21, 2022.
- Centers for Disease Control and Prevention. Control of Smoke From Laser/Electric Surgical Procedures. 1996. DHHS (NIOSH) Publication Number 96-128. Accessed January 21, 2022. https://www.cdc.gov/niosh/docs/hazardcontrol/hc11.html.
- Vitruk P. ANSI Z136.3 Standard Doesn’t Cover Safe Removal of Laser Plume Dispersed by Water Spray. Dent Today. 2020. https://www.dentistrytoday.com/standard-doesn-t-cover-safe-removal-of-laser-plume-dispersed-by-water-spray/. Accessed January 21, 2022.
Stay Relevant With Implant Practice US
Join our email list for CE courses and webinars, articles and mores